The adherence of worms to standard plastic tips makes accurate dispensing difficult. While using glass tips can overcome this problem, their application is limited when sterility and frequent tip changing is needed, for example, when performing liquid culture RNAi screens using 96-well plates (Lehner et al., 2006). RNAi screen protocols often require ~10 L1 worms to be dispensed into each well of a 96 well plate, however using standard P200 tips with worms in M9 alone resulted in an average variance of ± 6 L1 worms per well. We found that worms suspended in M9 with Triton X-100 decreased this adherence, resulting in an average variance of ± 2 L1 worms in each 10µl volume.
Both Triton X-100 and Tween-20 can be used to decrease this adherence to standard P200 tips. Since autoclaving either of these reagents is not recommended, sterilization can be carried out via filtration using a 2µm filter. The lower viscosity of Triton X-100 over Tween-20 greatly aided filtration and sterility tests showed no associated contamination. We found that using concentrations of Triton X in M9 ranging from 0.1% to as low as 0.01% proved to be effective in decreasing worm adherence throughout our assay. No negative issues regarding the growth or progeny of worms were observed when compared to a control plate containing no Triton X. Therefore this method could serve useful when applied to protocols that require consistently small numbers of worms in large repetitions.
References
Lehner B, Tischler J, Fraser, AG. (2006). RNAi screens in Caenorhabditis elegans in a 96-well liquid format and their application to the systematic identification of genetic interactions. Nat. Protoc. 1, 1617-20.
A simple yet effective method to harvest worms from a liquid culture
Although it is easy to grow worms in large quantities in liquid medium, harvesting worms is troublesome, especially when the culture is big. There are several options to collect worms (Hope, 1999). Centrifugation is the most typical procedure, yet in many cases the residual bacteria supplied as food in the culture is also precipitated and is hard to get rid of. A sucrose floatation step to obtain a pure worm sample is effective but difficult to scale up. An alternative way to harvest worms is to chill the culture flasks on ice, allow the worms to settle, then aspirate off the supernatant. This is easy and effective, but not all experiments tolerate a prolonged chilling step. Another harvesting option is to collect worms with mesh, which may be hard to keep sterile.
We use a Nalgene® separatory funnel (#4300), which is made of polypropylene with a Teflon TFE stopcock. Worms hardly stick to it. Although the funnel is autoclavable (stopcock is not autoclavable), we use ethanol to sterilize it. An 8-cm tripod (inner diameter) works well with both 500 ml and 1000 ml funnels. If you fully open the stopcock, the worms will come out within 2 seconds. The worm pellet should be pure. If you want it to be extra pure, you can repeat the steps with new buffer.
Figures
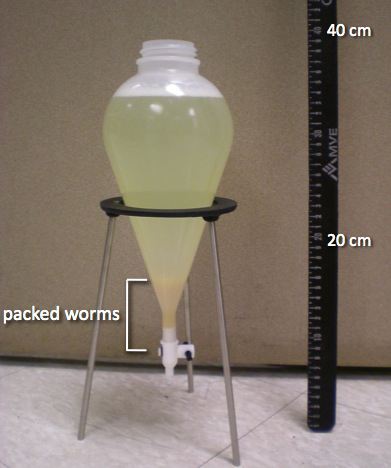
- Place a separatory funnel on a tripod.
- Close the stopcock.
- Pour the liquid culture into the funnel.
- Allow worms to settle for ~10 min at room temp.
- Gently open the stopcock over a collecting tube.
- Close stopcock when you have collected all worms.
References
Hope, IA. (1999). C. elegans. A practical approach. (New York: Oxford University Press).
Few gene expression differences between C. elegans grown in liquid versus on plates
The two major methods for growing C. elegans are liquid and plate culture. Differences in oxygenation and other environmental variables could affect many experimentally relevant biological processes, such as metabolism, cellular structure, and gene regulation. Worms on solid support move with deliberate sinusoidal movements, while worms in liquid thrash rapidly and almost constantly, often appearing elongated. We investigated differential gene expression under these two commonly used growth conditions.
Embryos were isolated by bleaching from adults grown for several generations on plates. After hatching in M9 media, animals were placed either on plates seeded with OP50 or in liquid supplemented with HB101 under standard conditions. Both cultures were grown until worms reached young adulthood, approximately 48 hours later. Total RNA was isolated independently from each growth, differentially labeled with a fluorescent dye, and mixed directly for comparative hybridization to 4-plex NimbleGen DNA microarrays, which contained 72,000 probes, which covered all predicted C. elegans protein-coding genes (minimum 3 probes per gene). The experiment was performed in triplicate. Analysis of the expression data yielded surprisingly few genes with differential expression, about 1%. Overall, 176 genes were downregulated significantly (p<0.01; Student’s t) in liquid culture relative to plates, but of these only 102 genes were down two-fold or more. A total of 121 genes were upregulated (p<0.01. Student’s t) in liquid; of these, RNA corresponding to 50 increased by at least two-fold.
Surprisingly few genes directly associated with a stress response were differentially regulated in this experiment. However, the single gene most strongly upregulated in liquid is nnt-1, which encodes a mitochondrial NADP transhydrogenase, which protects against oxidative stress (Arkbladt et al., 2005). Other molecules associated with reducing oxidation are also upregulated in liquid culture, including thioredoxin, and two glutathione-S-transferases. As a class, however, the group of genes most differentially regulated encode collagens. A total of 12 collagen genes were downregulated in liquid culture relative to plates, while none are upregulated. Other genes affected include those encoding proteins involved in ubiquitin-mediated proteolysis, Ras signaling, transcriptional regulation, ribosome function, and multiple membrane-associated proteins.
The limited changes in relative RNA levels under different culture conditions provide increased confidence in comparisons made between worms grown in liquid and solid culture, and more generally between datasets obtained in different labs under slightly different culture conditions. Knowing which genes are most responsive to different culture conditions tells us how environment affects the animal, and should be of interest for comparison to other stress and longevity global datasets. The data is being prepared for formal publication, and is available via GEO accession number GSE21526.
Towards an automatic method for toxicity and pharmacological testing in C. elegans
In toxicity and pharmacological studies C. elegans are exposed to different compounds and the effects analyzed by observing worm viability or behavior. An alternative approach is to use locomotor activity as a readout, which has been instrumental for assessing compound toxicity (Dhawan et al., 2000). Data acquisition usually requires microscope observation and manual counting, which is time consuming and inconvenient for extensive compound screenings. We have previously developed an automated method to detect C. elegans movement in which swimming worms are detected as they cross through infrared microbeams (Simonetta et al., 2007).
We are currently adapting this methodology for high throughput analyses. We have developed a 384 channel apparatus and successfully recorded the behavioral changes produced by toxic compounds (Figure 1). The effect increases with compound concentration and is dependent on exposure time. Our “Worm Microtracker” might be useful for the community to develop easier and faster toxicity and paralysis assays, opening the possibility of performing high throughput studies in C. elegans.
Figures
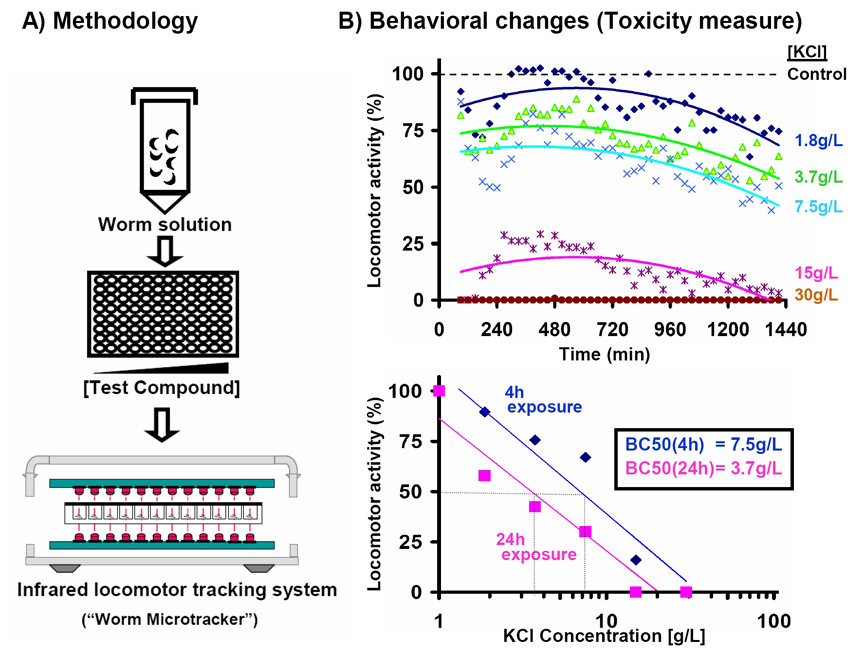
References
Dhawan R, Dusenbery DB, Williams PL. (2000). Comparison of metal-induced lethality and behavioral responses in the nematode Caenorhabditis elegans. Environ. Toxicol. Chem. 19, 3061-3067.
imonetta SH and Golombek DA. (2007). An automated tracking system for Caenorhabditis elegans locomotor behavior and circadian studies application. J. Neurosci. Methods 161, 273-80.
Measuring reactive oxygen species in C. elegans using DCFDA – a word of caution
Dichlorofluorescin diacetate (DCFDA) is a popular fluorescence-based probe for reactive oxygen species (ROS) detection in vitro and in vivo, and has been used for this purpose in C. elegans. DCFDA is first deacetylated by endogenous esterases to dichlorofluorescein (DCFH), which can react with several ROS to form the fluorophore DCF (reviewed by Halliwell and Gutteridge, 2007). The DCFDA assay in C. elegans is sometimes performed using lysed worms, following high intensity sonication. This process disrupts the outer cuticle and internal membranes, causing intracellular, as well as intraorganelle, contents to be released. Worm lysis will cause the release of transition metal ions such as iron. Free iron may participate in redox cycling to generate ROS, for example, by Fenton chemistry (Halliwell and Gutteridge, 2007). Therefore, apparent ROS levels detected in lysed worms may be un-physiological. However, DCFDA readily diffuses into cells where it undergoes deacetylation, and this allows ROS measurement in whole worms. If whole animals are utilized, cells are not disrupted and iron remains sequestered.
Using DCFDA and a synchronized worm cohort, we measured the amount of ROS in equivalent numbers of lysed and whole worms. The gradient of the linear regression curve (expressed as ΔRFU/Δmin) (Figure 1) is the rate of fluorescent DCF production. This gradient can be, very approximately, converted to the rate of H2O2-equivalent ROS production (nmol/min) by spiking known amounts of H2O2. It should, however, be noted that H2O2 itself does not oxidize DCFH, and hence, the free radical reactions resulting in increased DCF signal following H2O2 spiking are likely complex. Therefore, the calibration ratio can only be considered to provide an approximate estimation of ROS produced. Nevertheless, we found that the rate of H2O2-equivalent production was more than an order of magnitude faster in lysed (4.1×10-3nmol/min/worm) compared to whole animals (3.6×10-4 nmol/min/worm). Using the O2 consumption rate measured in the same worm cohort (0.018 nmol/min/worm), the % of O2 consumed leading to ROS generation can be estimated by dividing the rate of H2O2-equivalent production by the rate of O2 consumption. Using this approach, we found apparent ROS production to be around 23% for lysed worms, but only 2% for whole worms. Current estimates of in vivo mitochondrial ROS production range from 0.2 to 2% (Balaban et al., 2005). Assuming all ROS measured using the DCFDA assay to be of mitochondrial origin, ROS production is clearly overestimated by a factor of at least 10-fold, and may be as much as 100-fold, in lysed worms compared to whole worms. Therefore, when using the DCFDA assay, whole worms should be utilized instead.