Neuropeptides modulate neural circuits driving adaptive behaviors and key physiological processes such as reproductive behavior, metabolism, feeding, locomotion, and experience-based learning. With over 250 predicted sequences, bioactive peptides are by far the largest and most diverse group of neuromodulatory substances in the C. elegans nervous system (Li and Kim, 2010; Bargmann 2012,). Most of them are thought to function through the activation of G protein-coupled receptors (GPCRs) belonging to the Rhodopsin and Secretin classes. The C. elegans genome encodes at least 120 genes for neuropeptide precursors (FLP, NLP, and INS families), and recent studies have estimated a similar number of about 100 to 150 peptide GPCR genes (Frooninckx et al., 2012; Hobert, 2013). Although peptide signaling has undoubtedly diversified in the nematode lineage, many neuropeptidergic signaling systems share homology to vertebrate and protostomian systems (Mirabeau and Joly, 2013). Growing evidence implicates neuropeptides in many, if not all, behaviors in C. elegans. In contrast, only 23 C. elegans peptide GPCRs (isoforms included) have been deorphanized so far (Frooninckx et al., 2012), leaving more than 80 percent of all putative peptide receptors with unknown ligands.
Knowledge on functionally active neuropeptide-receptor couples and GPCR affinity is crucial to further expand our understanding of how neuropeptides function and modulate neural circuits. We are therefore undertaking a large-scale deorphanization initiative that aims at matching all predicted peptide GPCRs to their cognate neuropeptide ligand(s). The Peptide-GPCR project builds further on our combined reverse pharmacology approach (Figure 1), which in the past has enabled us to uncover several C. elegans neuropeptide systems – including cholecystokinin, pigment dispersing factor, and vasopressin/oxytocin related signaling, among others (Beets et al., 2012; and reviewed in Frooninckx et al., 2012). Both knowledge on natural peptides in tissue extracts and in silico peptide predictions are used to maximize the chances to identify a receptor’s cognate ligand(s). Through this combined approach, GPCRs are challenged with a collection of more than 260 peptides belonging to the established FLP and NLP families. The in vitro calcium mobilization strategy allows screening of these hundreds of peptides on all putative peptide GPCRs in a high-throughput manner.
The deorphanization of predicted C. elegans peptide GPCRs should provide a basis for furthering our understanding of nematode neuropeptide signaling and modulation. We aim to share progress on newly identified peptide-GPCR couples to the worm community, as we move down the random list of peptide GPCRs in the course of this project. We are working on a project’s website through which you can already help us prioritize peptides or receptor candidates. Community members are invited to steer the project’s progression via http://worm.peptide-gpcr.org.
Figures

References
Bargmann CI. (2012). Beyond the connectome: how neuromodulators shape neural circuits. Bioessays 34, 458-465.
Beets I, Janssen T, Meelkop E, Temmerman L, Suetens N, Rademakers S, Jansen G, and Schoofs L. (2012). Vasopressin/oxytocin-related signaling regulates gustatory associative learning in C. elegans. Science 338, 543-545.
Frooninckx L, Van Rompay L, Temmerman L, Van Sinay E, Beets I, Janssen T, Husson SJ, and Schoofs L. (2012). Neuropeptide GPCRs in C. elegans. Front. Endocrinol. (Lausanne) 3: 167, 1-18.
Hobert O. Neurogenesis in the nematode Caenorhabditis elegans (October 4, 2010), WormBook, ed. The C. elegans Research Community, WormBook, doi/10.1895/wormbook.1.12.2, http://54.165.141.74/.
Li C and Kim K. (2010). Neuropeptide gene families in Caenorhabditis elegans. Adv. Exp. Med. Biol. 692, 98-137.
Mirabeau O and Joly JS. (2013). Molecular evolution of peptidergic signaling systems in bilaterians. Proc. Natl. Acad. Sci. U. S. A. 110, E2028-2037.
Using the CRISPR/Cas9 system to target cis-regulatory sequences in C. elegans
The CRISPR/Cas9 system is rapidly becoming the genome-editing technique of choice in a variety of organisms (Pennisi, 2013; Frokjaer-Jensen, 2013). We recently applied the CRISPR/Cas9 module to target cis-regulatory elements in C. elegans.
We focused on a cis-regulatory region that is necessary and sufficient to drive lin-3 expression in the anchor cell (Hwang and Sternberg, 2004). This region harbours three regulatory sites in close proximity to each other: one NHR binding site, which is required for anchor cell expression, and two surrounding E-boxes on complementary DNA strands (Hwang and Sternberg, 2004). Interfering with these elements is expected to decrease lin-3 expression in the anchor cell and therefore lead to vulval hypo-induction as a phenotypic consequence.
Among current available methods, DNA-based delivery of the CRISPR/Cas9 complex has been reported to give rise to small deletions, like those presumably required to target specific cis-regulatory elements (Frokjaer-Jensen, 2013). We therefore used the plasmid vectors and protocol described in the first DNA-based CRISPR report in C. elegans (Friedland et al., 2013). To generate a pU6::lin-3 single guide RNA, named Ebox-2a, we used fusion PCR, as previously described (Friedland et al., 2013). However, instead of cloning first the PCR product into a vector, we PCR-purified and added it directly to the injection mix. We injected 15 N2 animals with a mix consisting of 40 ng/μl of eft-3::cas9 plasmid, 40 ng/μl of pU6::lin-3 sgRNA PCR product, 10 ng/μl of myo-2::GFP as co-injection marker and 10 ng/μl of pBluescript plasmid to reach a final 100 ng/μl concentration.
We recovered 4 injected animals segregating GFP-positive F1 progeny. From these 4 plates, we singled 29 F1 animals for further characterisation. We noticed that some transgenic F1 animals already showed a striking egg-laying defective (Egl) phenotype, which is the phenotype expected upon lin-3 reduction-of-function. However, when we sequenced Egl F1s, we found no deletion in the region of interest and the Egl phenotype was not heritable in the F2 generation. We conclude that, similar to other systems, Cas9 alone is sufficient in C. elegans to antagonise specific transcription factors that mediate transcription (Pennisi, 2013).
The progeny of some F1 animals isolated from 3 out of 4 P0 plates continued expressing GFP in the F2 generation and segregated back the Egl phenotype. We singled Egl worms and looked for heritable transmission in the subsequent generations. We identified four cases where the Egl phenotype was stable in the progeny and identified in all cases small deletions removing partly or entirely the NHR binding site and one E-box. We then sequenced the F1s and found that two deletions were already present in the F1 generation, whereas the remaining two occurred during the following generations.
We also designed another pU6::lin-3 sgRNA, Ebox-2b, targeting the nearest possible site to the previous construct, as defined by the G/A(N)19NGG rule. This construct was injected as a plasmid at 150 ng/μl concentration (300 ng/μl final concentration in the injection mix). We recovered 4 out of 15 injected animals segregating GFP-positive F1 progeny and singled 12 F1 animals for further analysis. Despite a frequent Egl phenotype in the F2, we found no heritable transmission of the phenotype and no molecular deletion in the F1s.
From the above experiments we draw the following conclusions:
1. sgRNAs can be injected as purified PCR products in C. elegans. This further simplifies the generation of targeting constructs for DNA-based genome editing, as it eliminates the single cloning step in the protocol.
2. When targeting cis-regulatory elements by CRISPR, the phenotypes of interest should be tested for their heritability.
3. We found evidence that CRISPR-mediated deletions can occur not only in the F1, but also in subsequent generations.
4. The efficiency of generating deletions can vary even when targeting directly adjacent genomic regions. Therefore, multiple sgRNAs should be tested for successful genome editing.
Figures
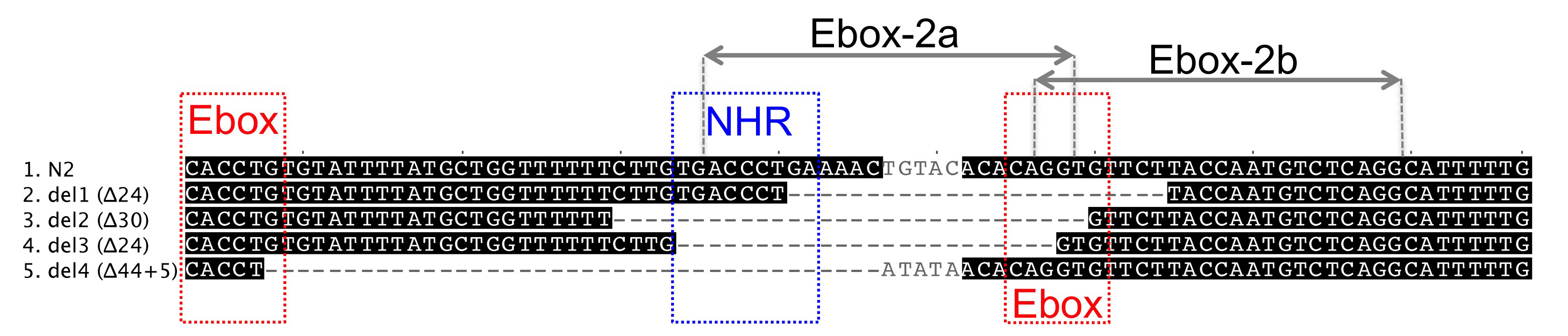
References
FriedlandAE, Tzur YB, Esvelt KM, Colaiacovo, MP, Church GM, and Calarco, JA. (2013). Heritable genome editing in C. elegans via a CRISPR-Cas9 system. Nat. Methods 10, 741-743.
Frokjaer-Jensen, C. (2013). Exciting prospects for precise engineering of Caenorhabditis elegans genomes with CRISPR/Cas9. Genetics 195, 635-642.
Hwang BJ, and Sternberg PW. (2004). A cell-specific enhancer that specifies lin-3 expression in the C. elegans anchor cell for vulval development. Development 131, 143-151.
High dose of D-glucose enhances cell growth in primary cultures of C. elegans cells
Primary cell culture techniques for embryonic and larval cells of C. elegans are well established (Christensen et al., 2002; Zhang et al., 2011). These cell culture methods utilize L-15 complete medium, which is prepared by the addition of FBS (fetal bovine serum) and sucrose to commercially available L-15 medium. L-15 medium is versatile as it does not include carbonate and hence does not require a CO2 incubator to maintain the optimal pH. Although L-15 complete medium is sufficient for the maintenance and differentiation of muscle cells and neurons in primary culture, there is little published information on the effects of varying culture conditions. Therefore, it seemed worthwhile to examine the comparative performance of other culture media.
We isolated primary cells from embryos using the established method (Christensen et al., 2002) and compared the growth of the cells in a number of commercially available culture media to which we added FBS. By comparison to other media, we found that GS medium, which is formulated for use in culture of mouse germline stem cells (Kanatsu-Shinohara et al., 2003), supported enhanced growth of the C. elegans cells (Figure 1A, B). Examination of the ingredients of GS medium suggested that its relatively high concentration of D-glucose was likely the principal factor for this growth enhancement effect. To determine whether this was the case, we added 6 g/L of D-glucose to L-15 complete medium and found enhanced cell growth. In medium with D-glucose, the diameter of the cells averaged more than 10 mm after two weeks of culture, while in standard medium they averaged about 5 mm (Figure 1B-E).
Typically, the cultures were initiated using 106 cells in 1.5 ml medium in 25 ml plastic culture flasks and incubated at 20°C. The addition of 6 g/L D-glucose caused about 10% of the embryonic cells to swell. The enlarged cells adhered weakly to the bottom of uncoated culture vessels and tended to form cell clumps. We cultured the embryonic cells using weekly addition of 500 ml fresh medium and a monthly change of the medium by collecting cells through centrifugation (1000 rpm, 3 min). With this periodic medium change, some of the enlarged cells survived more than 3 months.
Although, the cell population appeared homogeneous, examination of cultured cells from a transgenic strain with the chromosome integrated transgene Is[myo-2p::venus, rab-3p::NLS::mRFP] revealed the presence of several cell types. The fluorescent reporter genes Venus and mRFP, which were expressed under the control of the myo-2 and rab-3 promoters, respectively, showed at least 3 cell types were present: Venus+, mRFP+, and Venus-mRFP-. Expression of the Venus reporter gene was seen in only some of the enlarged cells, while that of mRFP was only present in small cells (Figure 2A-C). The cells showed BrdU incorporation only on the first day of dissemination indicating that they did not proliferate after enlarging (data not shown). Although the cell culture is not permanent, we expect that this method will provide cells that can be applied to a range of investigations.
Figures


References
Christensen M, Estevez A, Yin X, Fox R, Morrison R, McDonnell M, Gleason C, Miller DM 3rd, and Strange K. (2002). A primary culture system for functional analysis of C. elegans neurons and muscle cells. Neuron 33, 503-514.
Kanatsu-Shinohara M, Ogonuki N, Inoue K, Miki H, Ogura A, Toyokuni S, and Shinohara T. (2003). Long-term proliferation in culture and germline transmission of mouse male germline stem cells. Biol. Reprod. 66, 612-616.
Zhang S, Banerjee D, and Kuhn JR. (2011). Isolation and culture of larval cells from C. elegans. PLoS One 6, e19505.
Preserving histological samples of C. elegans
To compare antibody staining over time, one needs to have a method of archiving tissue samples. In general, however, C. elegans prepared for histology have not been archived for long periods. For example, worms mounted on slides sealed with nail polish and stored at 4°C (Wilson, et al., 2012; Duerr, 2013) last two weeks to a month (Duerr, 2013). After this time, significant tissue shrinkage occurs and fluorescent signals rapidly decay (Figure 1A). I have found that the polyvinyl pyrrolidone-based cryoprotectant known as “anti-freeze” (Tebbenkamp, et al., 2011) that is used to archive partially and fully processed mammalian tissues for long periods works well with histologically prepared C. elegans.
I prepared animals using the staining method of Finney and Ruvkun (Finney and Ruvkun, 1990) with antibodies against MEC-18 and MEC-2 or the 4′,6-diamidino-2-phenylindole (DAPI) probe against DNA or the actin-binding reagent phalloidin. Animals were then archived with or without anti-freeze for varying times. In contrast to sealing with nail polish no significant degradation of tissue morphology or fluorescence was seen for at least a year (Figure 1B-C). Similar results were observed with worm cell cultures. Thus, anti-freeze did not disrupt antibodies bound to their epitopes. In addition, animals expressing GFP and mCherry that were observed after several months in anti-freeze remained intensely fluorescent as were animals stained following long-term storage in anti-freeze. In general, the morphology of touch receptor neurons and other cells was indistinguishable in worms that were archived in anti-freeze compared to worms that were immediately analyzed. Moreover, morphology remained intact even after several freeze/thaw cycles.
To archive, mix 50 ml worm pellet and 500 ml anti-freeze (100 mM sodium acetate, 250 mM polyvinyl pyrrolidone (PVP), and 40% ethylene glycol, pH 6.5), and store at -20°C. The anti-freeze itself can be stored for years at 4°C or -20°C.
To recover worms for histology, pellet animals and wash 2-3 X with M9 buffer.
Figures

References
Duerr JS. (2013). Antibody staining in C. elegans using “freeze-cracking”. J. Vis. Exp. 80, doi: 10.3791/50664.
Finney M and Ruvkun G. (1990). The unc-86 gene product couples cell lineage and cell identity in C. elegans. Cell 63, 895-905.
Tebbenkamp AT, Green C, Xu G, Denovan-Wright EM, Rising AC, Fromholt SE, Brown HH, Swing D, Mandel RJ, Tessarollo L, and Borchelt DR. (2011). Transgenic mice expressing caspase-6-derived N-terminal fragments of mutant huntingtin develop neurologic abnormalities with predominant cytoplasmic inclusion pathology composed largely of a smaller proteolytic derivative. Hum. Mol. Genet. 20, 2770-2782.
Wilson KJ, Qadota H, and Benian GM. (2012). Immunofluorescent localization of proteins in Caenorhabditis elegans muscle. Methods Mol. Biol. 798, 171-181.
Collective behavior of C. elegans and C. briggsae starved L1 larvae
Working with starved L1 larvae of C. elegans and C. briggsae we noticed that these two species behave quite differently in starvation. First, C. elegans adults stop laying eggs after exhausting bacterial food, which eventually leads to internal hatching and bagging. C. briggsae do not show this behavior. This difference has been observed before (McCulloch and Gems, 2003). Second, at high enough density of worms, arrested C. elegans L1s aggregate on agar plates after several days of starvation (Fig. 1a). C. briggsae L1s do not form aggregates (Fig. 1b). Aggregation may serve several purposes ranging from decrease of surface to volume ratio and use of diffusible “public goods” to sharing information about quality of the environment. Third, survival of starved C. elegans L1s strongly depends on their density – the higher the worm density, the longer they survive (Fig. 1c) (Artyukhin et al., 2013). This holds true for starvation on plates as well as in suspension. Survival of C. briggsae L1s is independent of worm density (Fig. 1d). We believe that the aggregation and the density dependence are naturally connected as they seem to be correlated across Caenorhabditis species. Forth, starved C. elegans L1s release plenty of ascarosides (Fig. 1e,f). C. briggsae L1s release far less, both in variety (Fig. 1e) and in amounts (Fig. 1f). More than 100 ascarosides have been found in C. elegans (von Reuss et al., 2012), and although we do not know physiological functions for many of them, functions of the ones that we do know and the high degree of structural conservation in other nematodes suggest that ascarosides constitute a vital part of nematode chemical language. Hence, we interpret the data in Fig. 1e,f as suggesting that C. elegans L1s “talk” more with each other in starvation than C. briggsae. Finally, C. elegans is one of a couple Caenorhabditis species susceptible to environmental RNAi (Winston et al., 2007), which has been speculated to play a role in communication between organisms (perhaps, conspecifics) (Whangbo and Hunter, 2008). Based on everything above, we speculate that in unfavorable conditions (starvation) C. elegans tend to become more social than C. briggsae. In variable and unpredictable environments genotypic fitness can be maximized either by reducing individual-level variance in fitness or by reducing between-individual correlations in fitness (or some combination of the two) (Starrfelt and Kokko, 2012). Aggregating or social species may choose to minimize individual-level variance by having a mechanism that helps them to adjust to unfavorable conditions, in part through collective behavior. Non-aggregating species may use dispersal as a strategy to minimize between-individual correlations. Based on this hypothesis we would predict that lack of aggregation and density dependence in C. briggsae implies that their starved L1s hardly ever find themselves at high density in nature and the optimal strategy for them is to disperse and actively look for food.
Figures
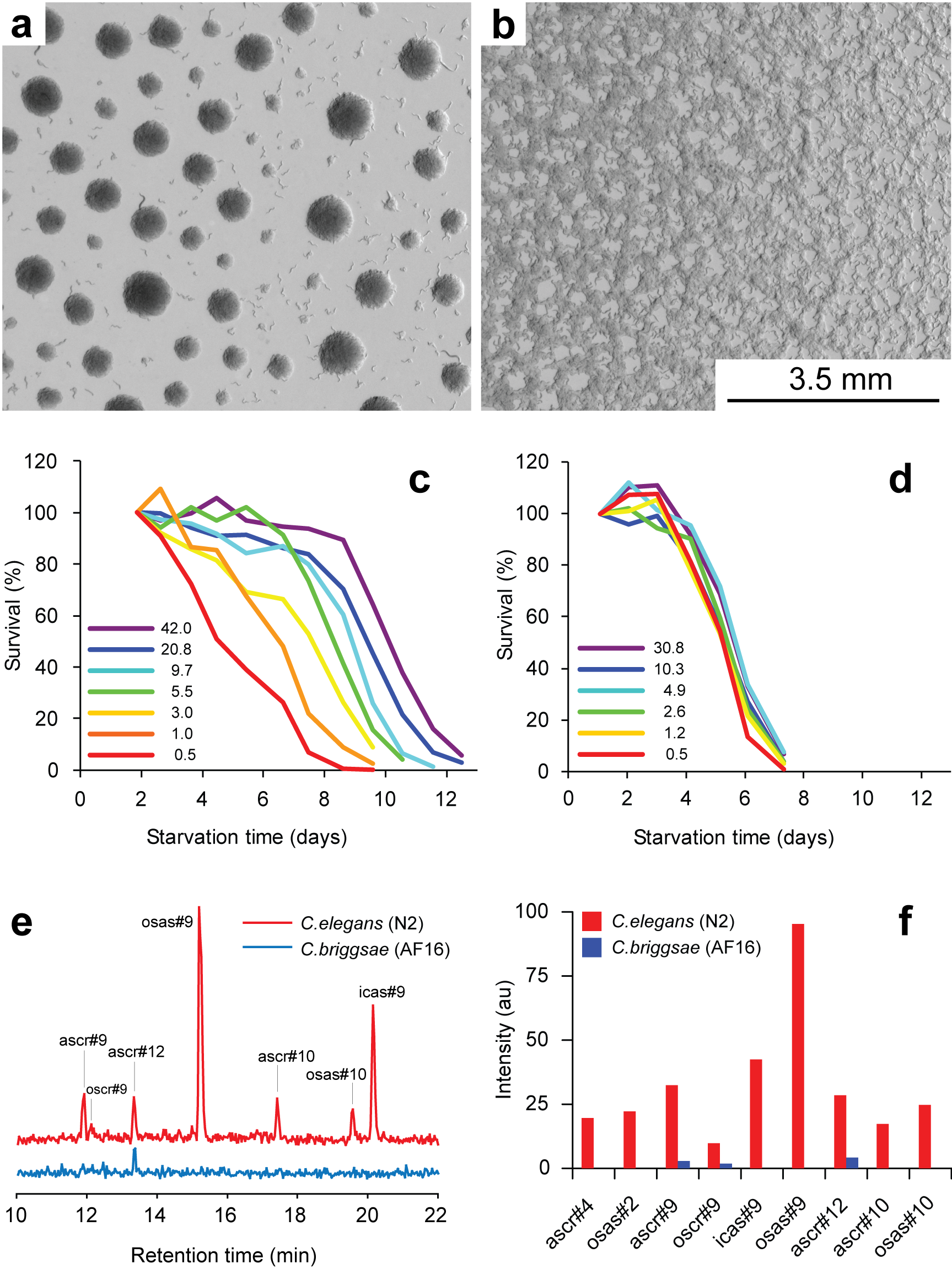
References
Artyukhin AB, Schroeder FC, and Avery L. (2013). Density dependence in Caenorhabditis larval starvation. Sci. Rep. 3, 2777.
McCulloch D and Gems D. (2003). Evolution of male longevity bias in nematodes. Aging Cell 2, 165-173.
Starrfelt J and Kokko H. (2012). Bet-hedging – a triple trade-off between means, variances and correlations. Biol. Rev. 87, 742-755.
Whangbo JS and Hunter CP. (2008). Environmental RNA interference. Trends Genet. 24, 297-305.
Winston WM, Sutherlin M, Wright AJ, Feinberg EH, and Hunter CP. (2007). Caenorhabditis elegans SID-2 is required for environmental RNA interference. Proc. Natl. Acad. Sci. U. S. A. 104, 10565-10570.
Winston WM, Sutherlin M, Wright AJ, Feinberg EH, and Hunter CP. (2007). Caenorhabditis elegans SID-2 is required for environmental RNA interference. Proc. Natl. Acad. Sci. U. S. A. 104, 10565-10570.